Walter Vlad
Manufacturing plays a key role for our pathway to sustainable manufacturing. While emerging disruptive technologies prove promising, their large-scale implementation is too slow to achieve climate targets. Stakeholders need to coordinate their efforts to incentivize technological innovation and facilitate its practical implementation. The future belongs to those with big visions.
The challenge of sustainable production
With the world’s total population likely to surpass ten billion by 2100 [1] and the desired increase in standard of living, the global production systems for food, goods and services are set to meet considerable challenges. Total consumption will increase while the environmental and socioeconomic consequences of climate change need to be limited. The Sustainable Development Goals [2], adopted by the UN in 2015, define numerous objectives that the member countries have agreed to aim for. The key goal of limiting the increase of global average temperatures to less than 1.5°C by the end of the century compared to the pre-industrial average requires a drastic reduction of greenhouse gas emissions and societal transformation.
By now, many countries have committed to achieving net-zero emissions by 2050 to prevent crossing irreversible tipping points for climate. The phase-out of fossil fuels from the economy, commonly dubbed decarbonization, is a widely acknowledged necessity, and emerging as a new paradigm to govern policies and industrial development for the foreseeable future. As many industrial processes and products are based on coal, oil and gas, the transition to a decarbonized society will entail drastic changes to business and society. With the common goal defined, the upcoming decade will set the course for the future as technology and policy roadmaps are being established. To challenge net-zero emissions by 2050, collaboration from all stakeholders involved is required.
On an abstract level, bringing humanity’s business practices to sustainable levels can be done via three levers: lowering demand for products and services, implementing material cycles with minimal resources wasted, and deploying innovative new technologies. In the following, I want to illustrate how the latter two steps may look like in the field of manufacturing.
Manufacturing between tradition and new opportunities
The manufacturing sector represents a crucial part of the equation to achieve a net-zero carbon global economy. Obviously correlated to several of the SDGs, its importance is much higher: manufacturing is closely intertwined with many aspects of daily life.
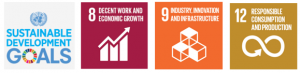
Figure 44.1 – The obvious but not the only Sustainable Development Goals related to manufacturing.
Look around the room you are sitting in. There is a high chance that the majority of objects catching your eye have been manufactured. The screen you are looking at. The coat hanger for your jacket. The pen you’re clicking when bored. And even of those things that don’t look like it, many have come into contact with industrial machines. Modern tractors harvesting produce we eat every day. Oats processed and packaged on food production lines. The machines yarn is produced on before being weaved into clothes. If you patch holes in your t-shirt rather than buy a new one, the needle you will use is one of millions that pass the same production line every week.
Despite having made considerable advances in efficiency over time, many production processes still rely on fossil fuels. In products that use the majority of energy in their active use phase, high manufacturing quality can lead to lower friction losses and a significantly increased energy balance. To allow for these benefits, precision manufacturing generally requires significantly higher specialized knowledge, efforts and costs. However, humanity has historically been able to recognize areas of improvement and come up with ingenious ideas to solve technological challenges. There already are numerous promising innovations in development that will provide advantages over existing alternatives.
Assessing the production of goods entails not only the manufacturing process and active-use phase, but the entire life-cycle including sourcing of raw materials, distribution and end-of-life considerations. As we substitute fossil-fuel-based products with green ones, the manufacturing process will require more attention to improve overall CO2 and natural resource balances. Like with every other sector, a decarbonized society will be out of reach without decarbonizing individual manufacturing processes as well. In the meanwhile, some carbon-intensive processes currently still provide advantages compared to electrified alternatives. Electric discharge machining processes, for example, can produce complex geometries for press tools that currently cannot be manufactured to the same quality using other processes, but requires the workpiece to be submerged in oil tanks for maximum surface quality and processing speeds. There are arguments that the increased efficiency of products based on such environmentally-damaging processes is justified if it leads to massively lower energy consumption in the use phase. Aiming for a zero-carbon industry, however, requires eliminating the use of non-synthetic fossil fuels wherever they are not strictly necessary. Manufacturing companies need to focus on finding true net-zero technologies.
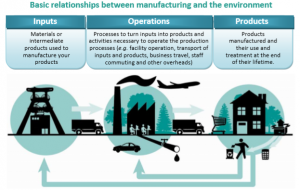
Figure 44.2 – When assessing the sustainability of a product or company, the entire life-cycle must be considered.
A major challenge regarding decarbonization of industry is the high degree of price sensitivity in manufacturing. When we say that money rules the world, this holds especially true for manufacturing. In a globalized world, raw materials, semi-finished products and components can be sourced and substituted relatively easily. The current Corona crisis shows how much our logistics and value creation chains are intertwined. This means that generally the cheapest option is chosen irrespective of other considerations.
While there are promising revolutionary innovations, they often come from players not fully established in their industries. We can see it particularly in Germany, where car manufacturers represent a large economic influence with many jobs in its wake from manufacturers to suppliers. They have a market lead in combustion engines, but not in the other, newer technologies. Keeping combustion engines alive as long as possible allows them to retain their competitive advantage. While part of the earnings is used to invest in development of electric and fuel cell vehicles, the reliance on combustion hinders a faster transition. The highly specialized KMUs (small and mid-size companies) that have historically developed off the strong automotive industry and provide a strong backbone of the German and Swiss manufacturing industry, encounter similar challenges. Their selling proposition has often been world-leading manufacturing technology for combustion vehicles. As quality depends largely on specialized knowledge acquired through extensive experience improving on practices, switching industries represents a large uncertainty. Nevertheless, the opportunity exists to become pioneers of cutting-edge sustainable technologies that will be highly requested in the future. The manufacturing of fuel-cell drives, for example, has some similarities with that of combustion engines, and allows for a transfer of existing knowhow. Other fields in rapid development, such as chemical engineering, allow to become leaders in a fast-growing market.
Aside from considerations of sustainability and electrification of processes, some other current trends in manufacturing shape up to cause long-lasting change. The popular term Industry 4.0 denotes the attempts to make use of advanced data analysis, monitoring and AI capabilities, which help monitor processes, identify improvement possibilities and optimize process parameters according to operating conditions. These approaches are excellently suited for electrification of machines and processes. Contrary to combustion motors, electric drives can be monitored with sensors and controlled easily. Another trend towards individualized and traceable products can also be observed.
To illustrate what manufacturing may look like in the coming decade, I want to mention two project examples.
HYBRIT: The Hydrogen Breakthrough Ironmaking Technology project (HYBRIT) by three Swedish companies researches the prospects of replacing coke in steel production with hydrogen. Hydrogen generated using renewable energies allows for steel produced with close to zero emissions, which is crucial as the worldwide production accounts for roughly 8% of direct emissions from the global use of fossil fuel [4]. Many similar projects aim to decarbonize industrial processes with hydrogen or other electrical alternatives. Even neglecting sustainability considerations, many processes can be made more efficient this way. This offers an enormous potential, as evidenced by the fact that the HYBRIT project alone is estimated to reduce Sweden’s CO2 emissions by up to 10% [5].
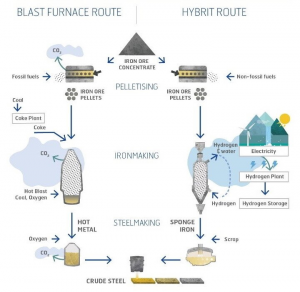
Figure 44.3 – Replacing coke with hydrogen in steel-making is a big step towards decarbonizing this process.
Toyota: In its Toyota Environmental Challenge 2050 [6], the Japanese car-maker defines objectives in agreement with the SDGs to make its entire operation carbon-neutral, focusing not only on the life-cycle emissions of manufactured cars but also on zero-emission plants and minimizing resource usage based on closed material cycles. While most manufacturers will follow suit, the initiative is encouraging, especially considering that Japan’s industry has long heavily depended on oil and gas imports.
Despite these attractive prospects, neither AI nor electrification are sensible or even possible for all processes. Where this is not possible, it is necessary to research how the problem may be solved using other approaches.
This brings us to the next question: How can we speed up the development of sustainable technologies and products?
Enabling the transition to sustainable manufacturing
The trend to sustainable manufacturing will over time evolve into a fundamentally new approach to engineering. Human innovation, and with it societal developments, are developing at a rate faster than ever before. Many of the necessary building blocks have already been established. Chances are, however, that a shift in technology innovation and consumer effect driven purely by market characteristics will be nowhere fast enough to achieve the climate goals agreed upon. When faced with the choice to stay with an existing custom or switch to a slightly better one, most actors tend to avoid the switch to reduce overhead. This is known as the lock-in effect, and can generally only reliably be mitigated by making the switch more enticing to the customer. Support from all sectors is needed to accelerate development and wide-scale roll-out of sustainable technologies. Measures that influence prices are arguably the most effective in influencing supply and demand. Such levers include reducing R&D costs through subsidies, incentivizing sustainable production of sustainable products and implementing taxes on less environmentally-friendly competing products.
Some challenges for industry actors have been previously mentioned to illustrate why many are slow to jump on the sustainability train. Most importantly, manufacturing companies must adopt sustainability as a core value so that sustainably produced products become available for all customers. In industry, goods are only offered if market demand justifies a price higher than the total costs of the manufacturer. This needs an environment in which the sustainable option is also the most attractive, or in other words, the cheapest option. It is necessary to incentivize research on promising sustainable technologies so that they can be deployed as quickly as possible. This can be done by funding research institutions to do fundamental leg-work that manufacturing companies then can expand on in their own R&D departments, supported through subsidies. Currently, unclear business prospects make companies and investors wary to fund the required startup costs in too many sectors, stunting the possible advances.
Universities and other research institutions also play an important role. Technological breakthroughs are commonly achieved in university labs or computers before being adapted by industry. Incremental improvements to existing manufacturing processes occur naturally in application and in part also at labs. Inventions and discoveries made at ETH have changed the world. While a high standard, we need to aim for better in the future. Knowledge is power; it is all the more necessary but also powerful in the pursuit of a sustainable society. The other, perhaps even more important, task of universities is to educate their students, to enable them to think critically and care about society’s challenges. While my experience as a mechanical engineering student may be different from that of many others in this course, it has showed me that sustainability is not often presented as an ethical goal by itself in the face of climate change, but rather as a secondary objective or even a nuisance. Decision makers passionate about the long-term consequences of climate can positively influence the direction of industry.
Key to enable fast progress on sustainable technologies and coordinating efforts are policies. Besides the important role of regulating business behavior, they are equally needed to support new developments. At the moment, consequences of greenhouse gas emissions and resource use are decoupled from the originator. Pricing them fairly to account for the true socioeconomic damage caused will increase prices of unsustainable goods. While attempts at setting appropriate prices exist, they need to be carefully considered. CO2 emissions certificates that correspond to the cost of sequestrating it in direct carbon capture (DCC) storage are not sufficient, as the technology is not yet established for large-scale operations and does not incentivize clean production enough if the price is low enough. Further, such certificates currently account only for one issue of exploitative industries, greenhouse gas emissions. Mining of minerals crucial for technological developments, such as bauxite, cobalt or nickel, also needs to be accounted for. An integrated approach to assess the environmental impact of industry actors should urgently be established in the near future. To introduce binding environmental management and accounting standards similar to, but exceeding the scope of ISO 9001 (quality management) and ISO 14000 (environmental management), political efforts are indispensable.
While meeting strong opposition from industry, political institutions and governments can certainly induce big changes to current business practices. Recently, regulations by the EU and China unilaterally putting limits on average fleet CO2 emissions on a relatively short timescale has forced car manufacturers to adapt. Pioneers in policy-making as in industry can force entire industries to adapt, for which the automotive industry is a nice (if disappointing) case study.
In order to get an idea for the impact of various policy measures, several interesting tools exist. The interested reader may check out tools like En-ROADS Simulator [7] and Energy Policy Simulator [8] to play around with models that visualize the quantitative impact of political measures like subsidies, carbon taxes and technology improvements on target variables related to the climate debate, such as projected temperature rise. While the underlying models are mostly top-down approaches and thus lack nuance in details, they allow a valuable insight in the importance of policy making. With increased collaborative research effort, they can be improved and serve as a basis for informed policy-making. Crucial projects will be to make finer models for individual industries, such as for manufacturing as in the context of this article, to identify current weaknesses and levers for making industry sustainable.
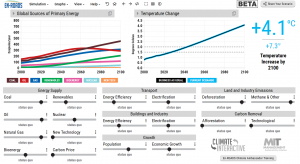
Figure 44.4 – The underlying model of the En-ROADS simulator predicts a temperature increase of more than 4°C by 2100 if the status quo is maintained without further measures. While nobody can accurately predict the future 80 years from now, it serves as an excellent entry into the realm of policy making.
Alongside subsidies, fundamental change also requires investors that finance the cost-intensive development of new technologies to fund promising sustainable business ideas. In the current dynamic market, many have become attractive investment opportunities, as seen by the numerous startups in automotive, artificial intelligence and automation applications. Banks, environmental insurers and investment funds must put an increasing focus on sustainability in their investment decisions and divest from fossil-fuel-intensive companies. The Government Pension Fund of Norway [9] is a pioneer in that regard, investing revenues from Norwegian fossil resource extraction into sustainable companies. Stakeholders should focus not on short-term dividends but long-term outlook and competitiveness in a new sustainable industry. While many investments won’t achieve big success, liberal funding is necessary to ensure promising ideas get funded.
Recently, numerous companies in highly competitive fields such as automotive manufacturing or autonomous driving have initiated joint ventures with competitors or new players. While often for financial reasons, the trend towards collaboration recognizes that pooling resources and sharing knowledge brings benefits in speed and quality of technology innovation. Sharing of experience manifested in best practices and data reduces the barrier of entry for new inventions. In the face of climate change, interdisciplinary research and collaboration in all fields needs to be encouraged despite perceived economic disadvantages. The growing output of open-access research in academia may make the transition to industry: So-called data jackets offer protected access to data for analysis purposes. Researchers at the University of Tokyo presented a concept they call the Market of Data: Entities in possession of data, be it from the manufacturing shop floor, process simulations or marketing, provide an interface over which customers can process data requests without directly accessing the underlying data [10]. Due to fear of providing competitors with an advantage, there is still work to be done to convince stakeholders of the benefits of collaboration. An important role belongs to industry associations to promote collaboration and channel the collective power of its member companies to drive sustainability forward.
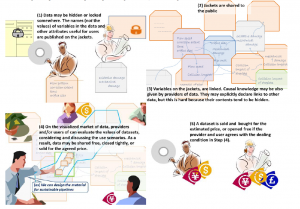
Figure 44.5 – Data jackets give the opportunity to work with data offered by other companies. Increased collaboration and data sharing has big potential, especially in applications like machine learning.
In academia, the creation of collaborative platforms to share research data and findings across disciplines can ease access to research and unlock the creative potential of researchers worldwide, especially considering the big interdisciplinary challenges of climate change and sustainability. Dataverses are open-source platforms for sharing research data, of which the Harvard Dataverse [11] is the most popular. For those interested in further approaches, the Github Open Science Training Handbook [12] provides a nice overview.
Whereas collaboration can speed up new developments, competition is fundamental to incentivize market-leaders to do the same. In the automotive industry, hydrogen and electricity-powered drive concepts have created a sector of economic importance with a relatively low entry barrier. This is even more pronounced in the run towards autonomous driving, where established car manufacturers compete or collaborate with rideshare providers, chip manufacturers and other technology companies. New technologies through the advent of digital services and artificial intelligence offer a great potential. Combined with established engineering best practices and increasingly popular approaches like modularity as bases for customizable products, the toolset is there for modern manufacturers to adopt sustainable manufacturing.
The final but most important prerequisite for a successful transition to sustainable production are we humans. We can change the way business works through informed consumer behavior. Changing priorities for a majority of the population will affect market characteristics and effect new policies, which in turn influence production and investment strategies. The resulting changes in prices reflect back on consumer behavior. Additionally, innovative business models like pay to use instead of buy to own can eliminate waste through unused products.
A perfect opportunity to question the predominant paradigms
Despite the apparently limitless possibilities through advancing technology, the social and economic systems need to evolve alongside technology. Pursuing the practical implementation of the Sustainable Development Goals and mitigating climate change requires fundamental changes to the underlying societal, economic and partly also moral systems upon which our behavior is founded. Placing priority on sustainable ways of life is not possible without also putting restrictions on previously unregulated practices. Because the changes required intrinsically challenge the existing systems, pushback from many stakeholders has slowed political and economic progress.
While dealing with the Corona virus and the loads it puts on our medical, societal and economic infrastructure is the priority at the moment, the involuntary hold on public life it has forced also gives us an unprecedented opportunity to revisit the paradigms that govern life. Everybody appears to be questioning the fundamental workings of our society.
Is working at the workplace necessary or shouldn’t it be substituted with working from home anyway? How can we increase resilience to our medical system? The previously rather marginalized idea of a universal basic income has now far-spread support. Does it make sense to be dependent on other countries in our logistics and production systems when it would be beneficial to have domestic capacities for production of essential goods now?
All these questions and more are being hotly debated now. This forced downtime we are experiencing now may help to also ask other fundamental questions. Is it worth going back to nuclear energy if it allows for quicker divestment from fossil fuels? Green growth or degrowth? A prominently featured opinion piece by Frans Timmermans and Bertrand Piccard [13] published last week raises similar questions on the society and industry of the future.
To learn and adapt to difficult problems requires courage, as well as admitting past errors and misguided strategies. Following the Great East Japan earthquake in 2011, researchers in Japan recognized that while the nuclear powerplant had been built according to standards, those standards did not account for security mechanisms under unforeseen external circumstances, such as the most powerful earthquake ever recorded in Japan [14]. In nuclear engineering, this sparked the emergence of the discipline resilience engineering in order to implement mechanisms to safely regain stable process conditions following unexpected external disturbances. Similar efforts have been adopted in several fields in Japan in recent years. The Corona virus provides us with the same opportunity to question current structures. It has also demonstrated that collaboration from completely different fields can be fruitful and quick when there is a will. Progress will be fast in the next years, and those that don’t actively try to participate in roadmaps to the future will have to deal with plans others have agreed upon.
The challenge of manufacturing
The transition to a sustainable global society presents us with numerous challenges that similarly include developing and developed nations, in which every sector needs to participate. The challenges in the domain of manufacturing are crucial to enable the pathway to a zero-carbon future. Because of long product development cycles, it is urgently necessary to put a focus on fundamental research and implementation of sustainable production technologies as quickly as possible. The transition to largely electrified manufacturing is underway and offers vast possibilities. To coordinate efforts, an unprecedented level of cooperation between experts, countries, companies, stakeholders and the public is needed.
By sharing knowledge and lowering the barrier of entry for all actors that can provide solutions, the deployment of new technologies can be accelerated. This includes funding research on sustainable industries. For those interested in more details on the topic of sustainable manufacturing, I can recommend the book Sustainable Manufacturing [15], which offers a detailed introduction to the topic highlighting a number of approaches and considerations.
The challenge of our lifetimes
In her excellent blog entry on the power of words, Stella Harper mentions the privilege of education and wide access to information we are lucky to enjoy. Similarly, we as ETH students are privileged to obtain a top-quality education. Among us are future researchers, professors, engineers, policy makers, CEOs, and most importantly, informed citizens that care about the direction we are heading in. There have been several fruitful events to popularize the topic of sustainability at our university, not least through this excellent lecture series.
When we retire several decades from now, the world will look very different from now. Different in a number of ways that would seem like far-fetched phantasies in this very moment. We won’t make a contribution to many of them, but we have been given the valuable opportunity to effect change. If we care about how the world will look like in fifty years, it is our duty to stay informed on the important developments on our path to sustainable society, to ask ourselves how we can contribute and effect meaningful change. For that, education and seeing the big picture of what is relevant and what isn’t is indispensable.
“Think big or go home” will be the motto in manufacturing as in many other sectors. Disruptors with groundbreaking ideas can shape the future. The challenge is difficult, but what is a challenge that’s easy to solve?
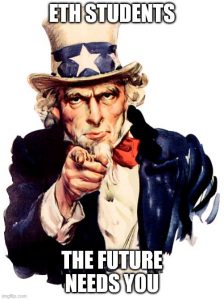
Figure 44.6 – Image generated using imgflip.com.
Sources:
Literature & web links
[1] United Nations, 2019: World Population Projection 2019. Data available at https://population.un.org/wpp/. Accessed 2020-04-22.
[2] United Nations, 2015. Sustainable Development Goals. Accessible at https://sustainabledevelopment.un.org/sdgs.
[3] OECD, 2020: OECD Sustainable Manufacturing Toolkit. Accessed 2020-04-21 at http://www.oecd.org/innovation/green/toolkit/48661768.pdf
[4] World Steel, 2020: Position paper. Steel’s contribution to a low carbon future and climate resilient societies. Accessible at https://www.worldsteel.org/publications/position-papers/steel-s-contribution-to-a-low-carbon-future.html
[5] HYBRIT. More information at http://www.hybritdevelopment.com/hybrit-toward-fossil-free-steel. Accessed 2020-04-21.
[6] Toyota: Toyota Environmental Challege 2050. More information at https://global.toyota/en/sustainability/esg/challenge2050/.
[7] Climate Interactive: EN-Roads Climate Change Solutions Simulator. Accessible at https://en-roads.climateinteractive.org.
Author’s note: Climate Interactive also hosts other policy simulators and simulation games on its website. I highly recommend checking them out.
[8] Energy Innovation LLC: Energy Policy Simulator. Accessible at https://www.energypolicy.solutions/
[9] Norwegian Government Pension Fund of Norway. More information at https://www.nbim.no/en/the-fund/about-the-fund/.
[10] Ohsawa et al. 2013: Data jackets for synthesizing values in the market of data. Procedia Computer Science, 2013, Vol.22, pp.709-716. doi: https://doi.org/10.1016/j.procs.2013.09.152.
[11] Institute for Quantitative Social Science, Harvard University: Harvard Dataverse. Accessible at https://dataverse.harvard.edu/
[12] Github: Open Science Training Handbook. Accessible at https://github.com/Open-Science-Training-Handbook/Open-Science-Training-Handbook_EN.
Author’s note: Chapter 2.7 provides an overview on collaborative research tools.
[13] Timmermanns, Piccard, 2020: Opinion Piece. Which world do we want after COVID-19? First published at: https://www.euractiv.com/section/climate-environment/opinion/which-world-do-we-want-after-covid-19/1455092/. Accessed 2020-04-18.
[14] Japan Meteorological Agency, 2013: Brochure: Lessons learned from the tsunami disaster caused by the 2011 Great East Japan Earthquake and improvements in JMA’s tsunami warning system. Accessed 2020-04-22 via http://www.jma.go.jp/jma/en/Publications/publications.html.
[15] Stark et al., 2017: Book. Sustainable Manufacturing. Cham Springer International Publishing. Accessible via ETH Library. doi: https://doi.org/10.1007/978-3-319-48514-0.
Figures
Fig. 1: Source: https://sustainabledevelopment.un.org/sdgs [2]. Screenshots taken 2020-04-21.
Fig. 2: Source: OECD Sustainable Manufacturing Toolkit, 2011 [3]. Accessed 2020-04-21.
Fig. 3: Source: hybriddevelopment.com [6]. Accessed 2020-04-21.
Fig. 4: Source: En-ROADS Simulator at Climate Interactive [7]. Screenshot taken 2020-04-21.
Fig. 5: Figure taken from Ohsawa et al. 2013 [10].
Fig. 6: Image generated using https://imgflip.com on 2020-04-22.
Media Attributions
- fig_1_sdgs
- fig_2_oecd_relationships_manufacturing_environment
- fig_3_hybrit_hydrogen_steel_production
- fig_4_en_roads_standard_setting
- fig_5_Ohsawa2013_data_jackets_market_of_data
- fig_6_imgflip_thefutureneedsyou